Updated April 13, 2022: Dr. Ye’s lab recently published “HYBRiD: hydrogel-reinforced DISCO for clearing mammalian bodies.”
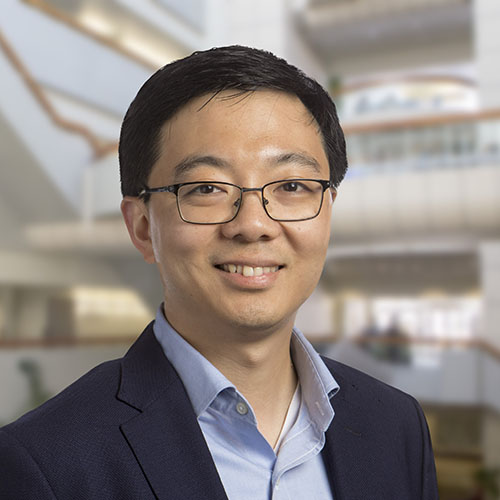
Learn more about the Ye Lab at the Scripps Research Institute.
LifeCanvas (LC): How did you arrive at your current neuroscience research interests?
Li Ye (LY): I have a background in peripheral metabolism, so I was interested in how tissues like fat and muscle communicate with the brain, and conversely how central brain regulation coordinates the function of these tissues. My current research is at an interesting interface between neuroscience and tool development, studying tissue clearing and brain mapping in the context of regulation between the brain and peripheral nerves.
In our forthcoming paper, my lab explored the benefits of combining organic solvent-based clearing methods like DISCO with aqueous methods like CLARITY and SHIELD in large peripheral tissues. The problem with solvent-based methods is that they quench fluorescence, while aqueous methods preserve fluorescence much more effectively. However, passive clearing with aqueous methods can be quite time-consuming. Our hybrid approach made a huge difference in peripheral organs, and we started to apply it to whole mice. While researchers typically use continuous perfusion to clear whole animals, our methods allow for full clearing of a whole juvenile mouse without any perfusion.
LC: How have LifeCanvas’ products helped you achieve your aims?
LY: In terms of reagents, EasyIndex is a lifesaver. We tried different refractive index-matching solutions in the past, but they were all either expensive or less effective. The RI = 1.52 EasyIndex works very well in peripheral tissues, especially bone and different connective tissues. This is a much easier and more economical solution for us than to make it from scratch.
The SmartSPIM microscope is really our lab workhorse. It’s very easy to use and robust, with unmatched speed and resolution – I personally think, compared to many commercial solutions, it’s probably one of the best systems out there. The support and customization from the team is remarkable. As we started ramping up our sample size, it became clear that we needed a much bigger chamber and updated software. Now we use customized chambers.
The flexibility of the optics and physical dimensions really make whole-body imaging possible. You can clear as large of a sample as you want, but without the proper optics, you can’t deliver the data, and thus you can’t fully take advantage of optical clearing. SmartSPIM allows us to easily and reliably see and generate large amounts of high quality data: this in turn allows us to do effective quantitative comparisons across different methods and transgenic animals. While our paper is mostly about clearing, the readout is fully dependent on the SmartSPIM microscope.
LC: How have new capacities for whole organ mapping influenced your research questions specifically, and basic science at large?
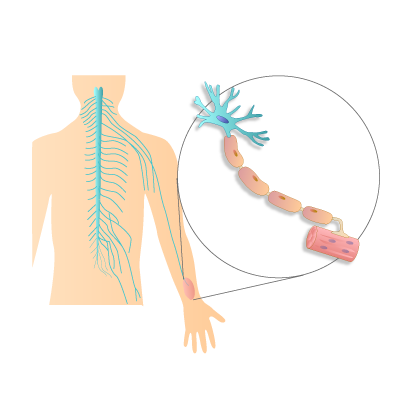
LY: In our future work, we want to understand the neurocircuitry of the whole body. We have just started to scrape the surface of this territory. People are advanced with whole-brain mapping, but when you move to peripheral organs, research is still based on methods like viral tracing and counting cell bodies. While the brain circuit has been increasingly well-characterized with modern methods, the peripheral circuitry has not been. Once a nerve travels outside the central nervous system (CNS), we don’t fully understand how it travels or branches, what organs it innervates, and whether there is overlapping organ innervation.
In basic research, these new mapping tools can also be applied in the development of transgenic animals. Cre lines, for instance, are supposed to be specific to certain cell types, but it’s very hard to know exactly whether the Cre mouse you generated actually has the specific expression pattern that you want. You hope something is only in the cerebellum, or the cortex, but it’s very tedious – almost impossible – to look through the whole body and see if there’s any leakage in other areas, like blood cells, liver cells, kidney cells. Now you can clear a whole mouse, image it under a light sheet microscope, get data in a week or two, and see every single cell in the body and where the trans gene is actually expressed. This will help you fully understand the phenotype and implications of a specific knockout, overexpression, or other transgenic model.
LC: Given the link between metabolism and neurodegeneration, do you have plans to further study neurological disorders?
LY: We are indeed interested in this. I think people are starting to realize that the brain is not an isolated computer controlling metabolism. We think that what is largely regarded as a one-way regulation from brain to metabolism is more dynamic, with things like fat and glucose affecting the brain as well. For example, ketogenic diets have been used as a medical approach for treating epilepsy, because we have seen that if you lower glucose in your diet and consequently lower your blood glucose, this can help moderate spontaneous brain activity.
There is an emerging realization that many so-called neurological diseases – such as Parkinson’s, Alzheimer’s, and more recently Multiple Sclerosis (MS) – have a lot of other components beyond the CNS. When you really look at the pathology of these diseases, you see that the gut contributes to Parkinson’s, viral infection may contribute to MS, diabetes or glucose dysregulation associated with aging may contribute to Alzheimer’s, and so on. As we develop better tools to look at neuroscience more holistically, we will continue to be surprised. The nervous system is affected by everything circulated throughout the body, including the blood, the immune system, and different pathogens.
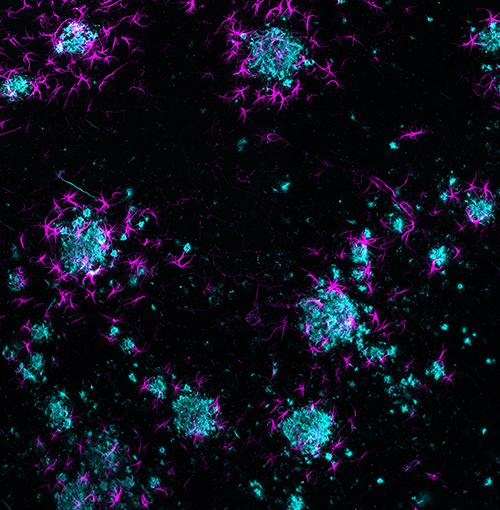
With these multi-system tools, including whole tissue clearing and imaging methods, we can better understand how different systems interact with the nervous system. Isolated methods such as cutting up tissue or measuring electrical impulses in a neuron may not fully capture all of these interactions, but looking at an intact system in 3D can really help us understand how they work.
LC: What other future questions would you be interested in pursuing with LifeCanvas’ technology?
LY: Beyond this paper, we also have more traditional brain-centered studies. Working with LifeCanvas’ whole-brain cFOS mapping, we look for new brain regions and circuits implicated in metabolic stress, such as what regions are affected by insulin and glucose in diabetic conditions. Eventually, we want to understand the whole circuit: how does a signal coming from the peripheral nervous system, such as fat tissue, get translated into the spinal cord and brain, and once it gets there, what does it do?
"Isolated methods such as cutting up tissue or measuring electrical impulses in a neuron may not fully capture all of these interactions, but looking at an intact system in 3D can really help us understand how they work."
Does the brain take this signal and generate different kinds of behavior, such as those implicated in learning and mobility? How are these decisions calculated in different brain regions? That’s the next step: understanding how and where the peripheral and central nervous systems are connected, and whether these interactions are different in mice under different metabolic conditions.
LC: Have you seen interesting applications of these methods outside of neuroscience?
LY: Our interdisciplinary collaborations with researchers such as chemical biologists and immunologists have shown promising uses of whole-tissue cellular resolution imaging. In exploring the potential of small molecule drugs, we can now look at how gene and protein expression responds to drug treatment across different tissues and cell types. You might think a drug works on the liver, but it might actually be working on the blood vessels instead of liver cells, or in the nerves of the heart instead of heart cells.
In a joint effort with a lab that studies different COVID models, we use whole-organ clearing to process humanized Ace2 transgenic mice infected with live COVID virus. This method is compatible with endogenous fluorescence as well as immunostaining. So we can stain for viral proteins in those cleared mice and, using whole-body imaging, see where in the chest the virus is binding. Researchers can take this information and look at different mutants or variants in different mouse models to see where in the lung or other systems the virus binds.