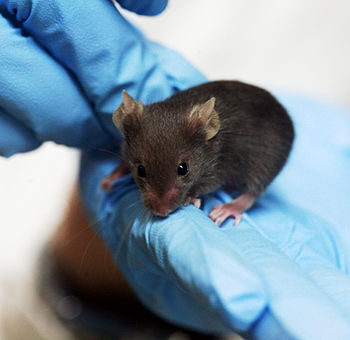
Rodents – particularly rats and mice – have long been used as models for biomedical research. The mouse model has risen to prominence in the last few decades, following the rapid advancement of tools for genetic manipulation since the publication of the first “Knockout” mouse by Thomas and Capecchi in 1987. These increasingly robust genetic tools, combined with remarkable similarities between the cellular architecture of the human and mouse brain, helped popularize mouse models in neuroscience research.
We spoke to Dr. Moriah Jacobson, Senior Manager of US Field Applications at Taconic Biosciences and expert in biomedical applications of mouse models, about the advantages and complications of using the mouse brain to study human neurological diseases. Dr. Jacobson also discusses how scientists are developing more effective mouse models for translational research.
LifeCanvas (LC): Why do so many neuroscientists study the mouse brain?
Moriah Jacobson (MJ): Mouse studies yield data necessary in the process of discovering new ways to diagnose, treat, and hopefully cure different diseases. Mice help us understand complex questions that we can’t tackle in humans for many reasons. For one, mice age much more quickly, so we can learn about diseases associated with aging, such as Alzheimer’s, at a faster rate.
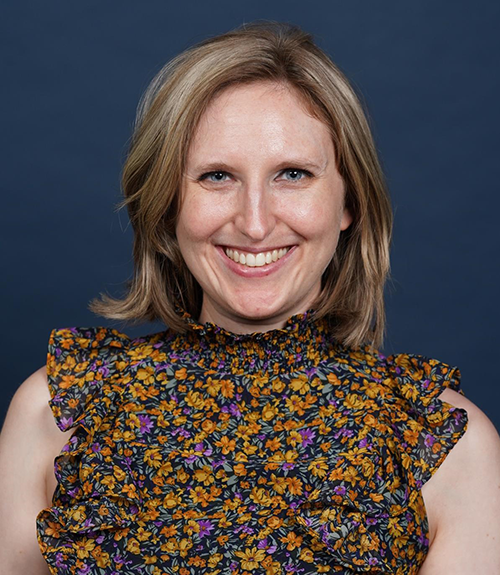
Second, animal studies allow you to closely control and manipulate environmental factors such as microbiome, diet, and stress levels, which can confound human studies. These confounds can result in correlative data, rather than the cause-and-effect relationships that researchers seek. Third, because their genome and genetics are relatively well understood, we can closely study how specific genes affect neurological function and disease. Additionally, many aspects of mouse neuroscience can translate to humans, such as learning, memory, cognition, social behavior, and pain.
LC: What complications arise with using mouse models for translational research?
MJ: Mice are definitely not mini humans. For example, APOE4 is a well-known genetic risk factor for Alzheimer’s disease (AD) in humans, but mice don’t have that form of APOE. So they need to be genetically modified to insert that human gene. Microglia are cells that also play a big role in AD; it’s been shown that microglia in the mouse brain may behave differently than those in the human brain. These are differences that you may not notice until you dive deeply into the mechanisms involved.
Another consideration is that you cannot directly test a behavior or condition such as depression or anxiety in mice. You can only test for depressive-like or anxiety-like behaviors. Sometimes, it can be difficult to determine if a mouse is in distress or pain in a way that translates to human conditions. In a related vein, you can’t exactly mimic human neurological conditions in the mouse brain. Researchers need to isolate a particular mechanism or phenotype instead of trying to encompass the entire disease state, which can actually decrease translatability.
LC: How can researchers improve the mouse brain model to increase relevance to human disease?
"Whole brain mapping gives you a much more complete picture, and is also more efficient than looking at each slice individually. Additionally, 3D data is less prone to bias."
MJ: Many neuroscience models have been critiqued because people can tend to take a “sledgehammer” approach to studying disease states. For example, in the AD field, many models combine disease mechanisms to develop a very strong phenotype. Then, when drugs are tested and found to be successful in these mice but unsuccessful in humans, people blame the mouse model. Perhaps a better approach would be to test one phenotype, one mechanism at a time.
At the same time, we are also over-simplifying other aspects of the mouse model. A lot of research is focused on inbred models, but the human population exhibits a lot of heterogeneity and genetic diversity. Scientists are beginning to realize that we may need to incorporate this diversity into our mouse models and study different genetic backgrounds.
Another approach consists of “humanizing” mice. Mice with humanized immune systems are commonly used in fields like immunology, oncology, and infectious disease. Neuroscientists are starting to incorporate humanized mice into their research as well. For example, since human and mouse microglia respond differently to AD plaques, researchers are beginning to study human microglia in the mouse brain. This allows us to see how a drug that will ultimately target human cells and receptors will interact with the same cells and receptors, just in a mouse.
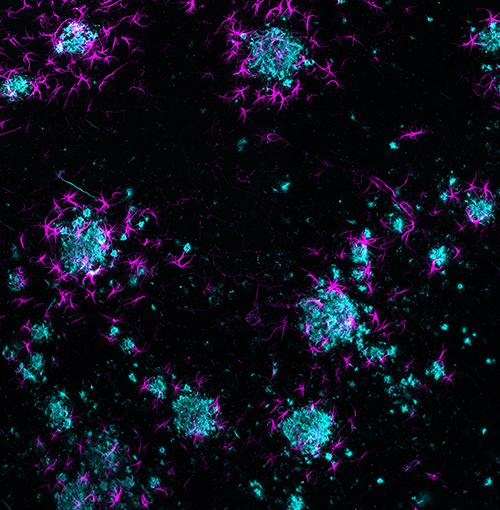
LC: How have technological developments such as 3D brain mapping affected research and development of mouse models?
MJ: Previous 2D approaches didn’t really allow you to see how different brain regions interact. Whole brain mapping gives you a much more complete picture, and is also more efficient than looking at each slice individually. Additionally, 3D data is less prone to bias. Working with 2D images, you typically have to choose an area to focus on, and find a representation of it. With 3D imaging technology, scientists are less dependent on an a priori hypothesis or the bias of human interpretation. The more sophisticated technology becomes, the more we learn, and the more we realize what we don’t know. I don’t think we’re ever going to be done asking questions and improving.